How can we tame transmission network oscillations?
What are transmission network oscillations, and why are they important? In this extended interview, Monash Grid Innovation Hub founding director Associate Professor Behrooz Bahrani, explains the ins and outs of this esoteric phenomenon.
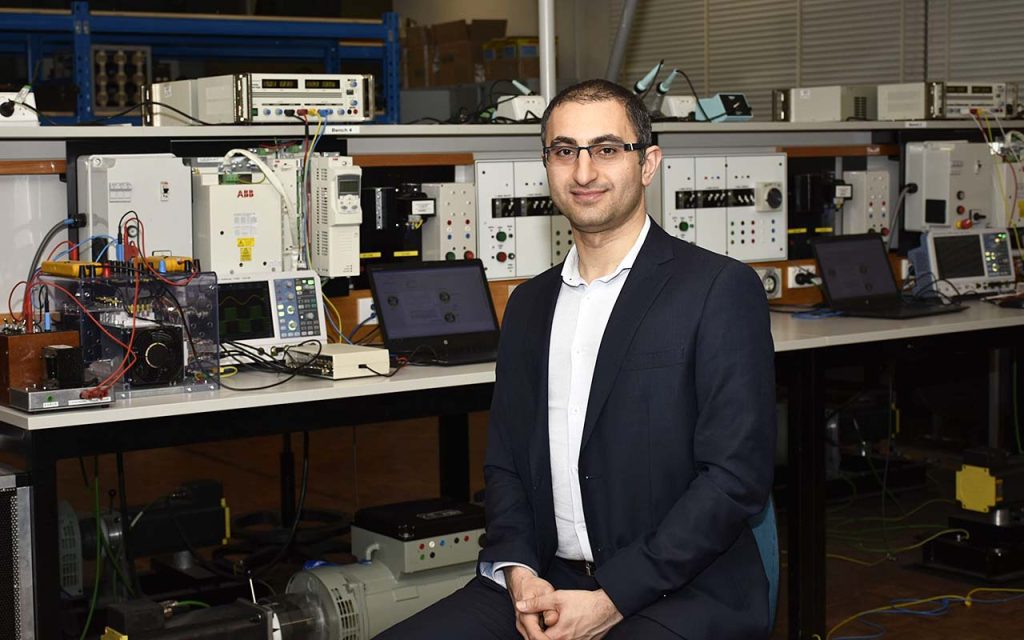
With today’s rapid growth in renewable energy sources, the stability of the nation’s electricity transmission networks is an area of increasing study.
Previously, much of the grid’s system strength and system inertia was provided by the large, rotating masses of fossil fuel generators. But now, with an increasing number of inverter-based resources, maintaining network stability has become more complex.
One of the trickiest problems is that of transmission network oscillations, which have the potential to lead to catastrophic system failures.
To help gain a better understanding of how inverters combine to produce oscillations, ARENA is supporting Monash University to develop a tool to investigate their root causes.
The tool is intended for use by the Australian Energy Market Operator (AEMO) and Transmission Network Service Providers (TNSPs), and will have applications for system planning, grid connections and operations.
ARENA is providing $499,774 funding to the $1.3 million project.
ARENAWIRE spoke to founding Director of Monash’s Grid Innovation Hub Associate Professor Behrooz Bahrani.
Q: What exactly are oscillations in an electricity grid network?
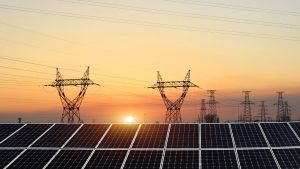
Well, to get a handle on oscillations, I think we need to rewind a bit.
There’s a big shift happening in our power systems right now. We’re adding a lot of inverter-based resources: think solar farms, wind farms, even large-scale batteries.
Now, before this, we relied on synchronous generators which were, to put it simply, straightforward with behaviours we really understood. But these new inverters? They’re a different ball game. They’re versatile, but their control systems can vary a lot depending on the vendor.
So, when all these differing systems interact, they might throw the network off balance. This is where those oscillations in frequency and voltage come into play. And in the worst-case scenario, the grid could become unstable.
Q: What kind of variations are we talking about in these oscillations?
Imagine this: under perfect conditions, you’d expect your household voltage to be a stable 230 volts RMS at 50 Hz. But in today’s evolving grid, things aren’t always so straightforward.
With the introduction of inverter-based resources, we’re noticing sub-synchronous and super-synchronous oscillations.
Now, these aren’t your typical oscillations from synchronous generators, which, as I mentioned, we’ve pretty much got down to a science. These new oscillations arise from control interactions between inverter-based resources and the system.
For instance, inverters bring their own control systems, and when they interact with the grid, it can result in these sub-synchronous and super-synchronous oscillations. What’s more, the potential instability in their control can magnify these effects.
The frequency, voltage, and even the waveform can vary, introducing these unique oscillation patterns.
Factors influencing these oscillations include the number and types of inverters, the resilience of the system, the characteristics of transmission lines, and the tuning of controllers. This becomes especially complex given that every inverter might come with its unique control philosophy, depending on its manufacturer.
Our project aims to dissect these issues, helping us understand and mitigate these oscillation patterns better.
Q: How damaging to an electricity grid can these oscillations be?
Here’s the crux: if these oscillations spiral out of control, they can tip the system into instability.
Sure, if they remain consistent and within limits, it’s like, “Okay, we can work with this.” But the moment they start amplifying, that’s when alarms should go off.
We might have to cut off a part of the network or, in dire situations, face a full-blown cascade failure.
Our aim is to catch these potential problems early, even before an asset joins the network. If we can identify a risk, we can loop in the vendor and sort it out before it becomes an actual problem.
And because the grid evolves, we need to stay on our toes, ready to adapt when an inverter starts acting up due to new changes.
Q: What is the tool that you are developing?
Ah, now we’re diving into the heart of it.
Typically, when we connect assets to the network, there’s a suite of tests we conduct – the [AEMO] GPS or Generator Performance Studies. These are largely time-domain analyses, which give us one piece of the puzzle. But there’s another crucial dimension we’ve been looking into: the frequency domain.
Our tool is fundamentally a frequency-domain tool. Instead of merely relying on time-domain studies, this tool focuses on the impedance of inverter-based resources and the grid they’re connecting to, all within the frequency domain.
By identifying and analysing these impedances, we can delve deeper into potential instabilities or interactions that might arise.
Think of it as a magnifying glass that can spot the sources of instability by assessing the interactions between the grid and the inverters in the frequency spectrum.
And the AEMO tests? They’re great, but our tool brings an added layer of depth and precision. By integrating this tool into platforms like PSCAD (Power Systems Computer Aided Design), we aim to offer grid operators and inverter designers a more comprehensive analysis.
In essence, it’s about pinpointing oscillations and potential issues by looking at the entire picture – both in time and frequency. This not only provides a clearer understanding but also speeds up the connection application process, ensuring that every piece of equipment behaves harmoniously with the network.
LIKE THIS STORY? SIGN UP TO OUR NEWSLETTER
Andrew Webster